About Us
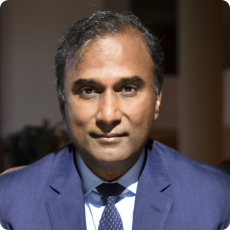
Founder
CytoSolve, Inc. was founded in 2011 by Dr. V.A. Shiva Ayyadurai, M.I.T. Ph.D. in Biological Engineering, the Inventor of Email, Fulbright Scholar, and world-renowned systems scientist. CytoSolve was a result of Dr. V.A. Shiva Ayyadurai’s M.I.T. Ph.D. research and a fulfilment of his life-long mission to enable human health and well-being by developing medicines and protocols, integrating Science and Tradition, Ancient and Modern, East and West.
How CytoSolve® Works
The Problem
The trillion-dollar pharmaceutical industry is in peril because of its medieval product development approach that is unable to predict toxicity and risk upfront in the development process. Meanwhile, the emerging market for supplements, herbs, and functional foods, while a potential alternative to conventional medical interventions such as drugs, vaccines and cell therapies, is producing products in an ad-hoc and unscientific manner. CytoSolve provides a revolutionary technology platform for addressing the major problems across both conventional and alternative industries by addressing a fundamental problem in the product development of pharmaceuticals, nutraceuticals, vaccines, supplements, functional foods, and cosmeceuticals: the inability to determine efficacy and toxicity, upfront in the product development process, without the need for animal testing and risk to humans during clinical trials.
The Solution
CytoSolve provides the world’s first computational systems biology platform for scalable integration of molecular pathway models to enable predictive and quantitative understanding of complex biomolecular processes and diseases to determine risk, toxicity, and efficacy UPFRONT in the product development process. CytoSolve’s technology platform is enabling innovative and visionary manufacturers to develop and deliver products to end-consumers that truly advance health and well-being, faster, cheaper, and safer. For example, CytoSolve is empowering the scientific analysis for the qualitative-quantitative determination of key parameters in the Certified C.L.E.A.N. and Certified R.A.W. certifications, that is supporting healthy product decisions by the end-consumer.